Ever wondered how a towering redwood tree gets water all the way up to its highest leaves, defying gravity day after day? Or why your houseplant needs regular watering? The answer lies in a fascinating, fundamental process called transpiration. It’s the plant’s way of moving massive amounts of water, but it comes at a cost. Think of it as a necessary consequence of breathing – plants need gases from the air, but opening up to get them means losing precious water. Let’s unpack this critical balancing act.
IB Biology B3.1.9: Transpiration – The Inevitable Cost of Gas Exchange
At its core, transpiration is the loss of water vapour from a plant, primarily through small pores on the leaves called stomata (singular: stoma), but also slightly through stems.
Why does this happen? Plants need to perform photosynthesis to create their food (glucose). This requires taking in carbon dioxide (CO₂) from the atmosphere and releasing oxygen (O₂). To facilitate this gas exchange, leaves are structured with:
Large Internal Surface Area: Spongy mesophyll cells inside the leaf have moist surfaces and are loosely packed, creating air spaces. This maximizes the surface area for CO₂ absorption.
Stomata: These adjustable pores on the leaf surface (usually the underside) allow gases to diffuse between the internal air spaces and the external atmosphere.
The Trade-Off: When stomata open to allow CO₂ in, water vapour inevitably diffuses out. This happens because the air spaces inside the leaf are typically saturated with water vapour (high water potential), while the outside air usually has a lower concentration (lower water potential). Water moves down this concentration gradient – from high inside to low outside.
Think of it like this: Imagine opening your front door on a cold winter day to let a friend in. You achieve your goal (friend enters), but you inevitably lose some heat from your house to the colder outside air. Plants face a similar dilemma with CO₂ and water.
Key Takeaway: Transpiration is fundamentally linked to the leaf’s structure, which is optimized for capturing CO₂ for photosynthesis. It’s an unavoidable consequence of needing to ‘breathe’.
The Mechanism: From Leaf Evaporation to Root Uptake
How does this water loss actually drive water movement through the entire plant? It’s a multi-step process driven by physical properties of water and differences in pressure.
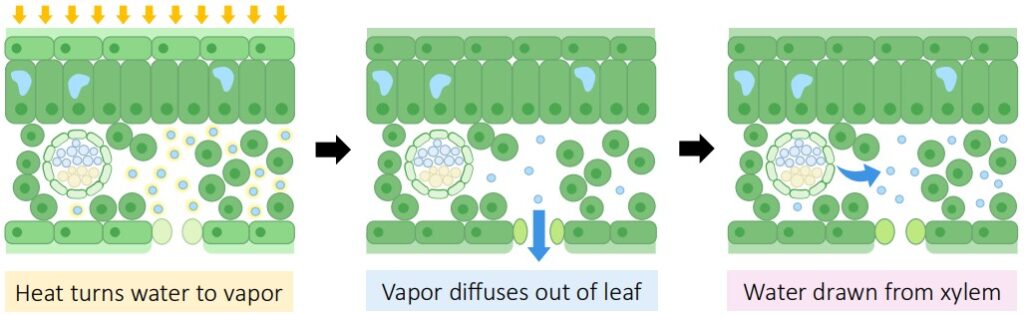
1. Evaporation in the Leaf
-
Sunlight energy absorbed by the leaf heats it up.
-
This energy causes liquid water coating the surfaces of the spongy mesophyll cells to evaporate, turning into water vapour.
-
This water vapour fills the internal air spaces.
-
When stomata are open, this vapour diffuses out into the atmosphere (transpiration).
-
Crucially: As water evaporates from the mesophyll cell surfaces, it lowers the water potential (the measure of free water molecules’ ability to move) in those cells. This creates a ‘pull’ or tension.
2. Generating Tension (Negative Pressure)
-
The water lost from the mesophyll cells needs replacing.
-
Water is drawn out of the nearest xylem vessels located in the leaf veins, moving into the mesophyll cells by osmosis and across cell walls via capillary action (water’s ability to move in narrow spaces).
-
This pull extends down the xylem, creating a continuous negative pressure, or tension, much like sucking liquid up a straw.
3. Root Uptake: Replenishing the Supply
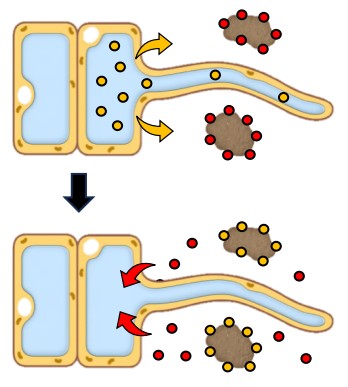
The tension generated in the leaves eventually extends all the way down the xylem to the roots.
Meanwhile, roots actively absorb water from the soil via osmosis. Osmosis is the passive movement of water across a semi-permeable membrane from an area of higher water potential (lower solute concentration, like dilute soil water) to an area of lower water potential (higher solute concentration, inside root cells).
How do roots maintain a low water potential?
Mineral Ion Absorption: Root cells actively pump protons (H⁺ ions) out into the soil using ATP (energy). These H⁺ ions displace positively charged mineral ions (like K⁺, Mg²⁺, Ca²⁺) that are loosely attached to negatively charged clay particles in the soil.
These freed mineral ions then diffuse down their concentration gradient into the root cells (this is sometimes called indirect active transport because ATP is used for the proton pump, which enables passive mineral uptake).
The accumulation of these mineral ions (solutes) makes the cytoplasm and vacuole of root cells hypertonic (lower water potential) compared to the soil water.
Result: Water naturally flows into the root cells via osmosis.
Optimizing Uptake:
Root Hairs: Tiny extensions of root epidermal cells vastly increase the surface area available for water and mineral absorption.
Aquaporins: Special protein channels in cell membranes facilitate faster movement of water molecules.
B3.2.7 & B3.2.8: Mass Flow via the Xylem Highway
The Cohesion-Tension Theory
The movement of water from roots to leaves is explained by the Cohesion-Tension Theory. This relies on three key properties of water and the structure of the xylem:
-
Cohesion: Water molecules are polar and form hydrogen bonds with each other. This makes them ‘stick’ together, forming a continuous, unbroken column within the narrow xylem vessels. Like beads on a string.
-
Adhesion: Water molecules are also polar and form hydrogen bonds with the polar molecules (cellulose) in the xylem vessel walls. This ‘sticking’ to the walls helps counteract the force of gravity, preventing the water column from breaking.
-
Tension: As water evaporates from the leaves (transpiration), the cohesive forces pull the entire column of water upwards, from roots to leaves. This pull creates tension (negative pressure) within the xylem.
Mass Flow: The combination of cohesion, adhesion, and tension results in the mass flow (or bulk flow) of water – the movement of water molecules together in one direction – up the xylem. This is a passive process driven by the water potential gradient created by transpiration, not by metabolic energy expenditure within the xylem itself.
Think of it like this: Imagine a very long, thin straw reaching from a glass of water (soil) up into the air. If you could somehow cause water to evaporate continuously from the top opening of the straw (leaf), the cohesive forces between water molecules would pull the entire column of water up the straw (xylem) from the glass (roots).
Efficiency Note: Astonishingly, over 95% of the water absorbed by roots is lost through transpiration! Only a tiny fraction is used for photosynthesis and maintaining turgor pressure within cells.
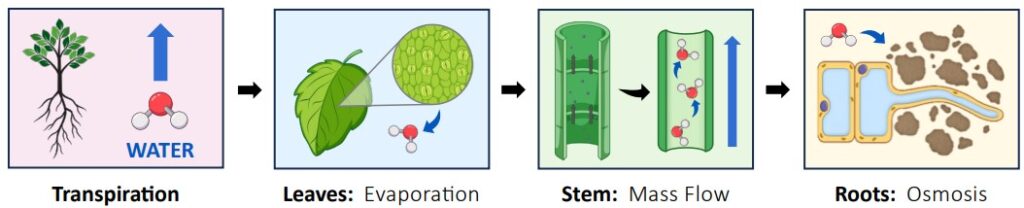
Xylem Vessel Adaptations for Water Transport (B3.2.8)
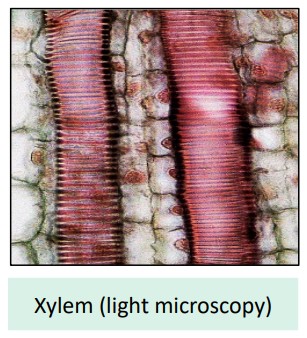
The xylem tissue is remarkably adapted for efficient water transport:
Hollow, Dead Cells: Mature xylem vessels (tracheids and vessel elements) are dead cells. They lose their cytoplasm and organelles, forming continuous, hollow tubes with no internal obstruction to water flow. Think of them as perfectly clear pipes.
Continuous Tubes:
Vessel Elements: Found mainly in flowering plants (angiosperms). These are wider cells joined end-to-end. Their end walls are perforated or completely removed, forming long, efficient pipelines called vessels. Ideal for rapid water transport.
Tracheids: Found in all vascular plants. These are narrower, tapered cells that overlap. Water moves between tracheids more slowly through pits (thin areas) in their side walls.
Lignified Walls: The cell walls are thickened with cellulose and reinforced with lignin, a complex polymer.
Strength: Lignin provides mechanical strength and rigidity, preventing the xylem vessels from collapsing under the strong negative pressure (tension) created by transpiration.
Waterproofing: Lignin helps waterproof the walls, keeping water within the vessel.
Pits: Non-lignified areas (pits) in the walls allow water to move sideways between adjacent xylem vessels or out into surrounding tissues if needed.
B3.1.10: Stomata, Guard Cells, and Regulation
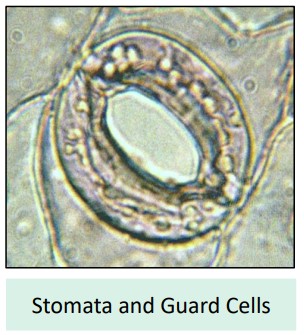
Plants aren’t passive victims of water loss; they can control it by regulating the opening and closing of their stomata.
Guard Cells: Each stoma (pore) is flanked by two specialized guard cells.
Turgor Pressure Control: The opening and closing mechanism depends on changes in the turgor pressure (water pressure) within the guard cells.
Opening: When guard cells actively accumulate solutes (like potassium ions, K⁺), their water potential decreases. Water enters the guard cells via osmosis, making them turgid (swollen). Their unique cell wall structure causes them to bow outwards, opening the stomatal pore. This is typically stimulated by sunlight (for photosynthesis) and low CO₂ concentrations inside the leaf.
Closing: When guard cells lose solutes (K⁺ ions exit), their water potential increases. Water leaves the guard cells via osmosis, making them flaccid (limp). They sag inwards, closing the stomatal pore.
ABA Trigger: Under conditions of water stress (e.g., drought), mesophyll cells release a plant hormone called abscisic acid (ABA).
ABA travels to the guard cells.
It triggers the efflux (outward movement) of K⁺ ions from the guard cells.
Water follows by osmosis, guard cells lose turgor, and the stomata close, conserving water.
Stomatal Density (B3.1.10)
Definition: Stomatal density refers to the number of stomata per unit area of the leaf surface.
Variation: This density varies significantly between plant species and even within the same species depending on:
Genetics: Some species are inherently adapted to have more or fewer stomata.
Environmental Conditions: Plants grown in high light and low CO₂ might develop higher densities. Plants adapted to arid (dry) environments (xerophytes) often have lower stomatal densities, sunken stomata, or thick cuticles to minimize water loss, compared to plants in humid environments.
Factors Affecting Transpiration Rate
Several abiotic (non-living) environmental factors influence how quickly transpiration occurs:
Temperature:
Effect: Higher temperatures increase the rate.
Why: Increases the kinetic energy of water molecules, leading to faster evaporation from mesophyll cells. Also, warmer air can hold more water vapour, potentially steepening the diffusion gradient if the outside air isn’t already saturated.
Light Intensity:
Effect: Higher light intensity generally increases the rate (up to a point).
Why: Light stimulates stomatal opening to allow CO₂ uptake for photosynthesis. More open stomata mean more pathways for water vapour to escape.
Wind:
Effect: Increased wind speed generally increases the rate.
Why: Wind removes the layer of humid air (the boundary layer) that accumulates around the leaf surface. This maintains a steeper water potential gradient between the inside of the leaf and the outside air, promoting faster diffusion. Imagine blowing across a wet surface – it dries faster.
Humidity:
Effect: Higher relative humidity decreases the rate.
Why: Humidity refers to the amount of water vapour already present in the air. If the outside air is very humid (high water potential), the water potential gradient between the leaf interior and the exterior is smaller. This reduces the rate at which water vapour diffuses out.
FAQ: Transpiration Troubleshoot
It’s primarily a consequence of gas exchange (getting CO₂ for photosynthesis). However, the resulting water flow is essential for transporting dissolved mineral ions from the roots to the leaves and also helps cool the plant through evaporation.
Cohesion is water molecules sticking to each other (H-bonds). Adhesion is water molecules sticking to other polar surfaces, like the cellulose in xylem walls (H-bonds). Both are vital for maintaining the continuous water column.
The bulk flow of water up the xylem (mass flow driven by cohesion-tension) is passive, powered by the energy of evaporation (sunlight). However, the setup requires active processes: active transport is used in the roots to pump H⁺ ions out, enabling mineral uptake which creates the osmotic gradient for water absorption. Stomatal opening/closing also involves active transport of ions into/out of guard cells.
Plants in dry environments (xerophytes) often have lower stomatal density, sunken stomata, or hairs around stomata to reduce water loss. Plants in very humid or aquatic environments might have higher densities or stomata primarily on the upper surface. It’s an adaptation to balance gas exchange needs with water conservation challenges.
Primarily, yes – the main flow is unidirectional from roots to leaves. However, the presence of pits allows for some limited lateral movement of water between adjacent xylem vessels or out to nearby living tissues if their water potential is very low.